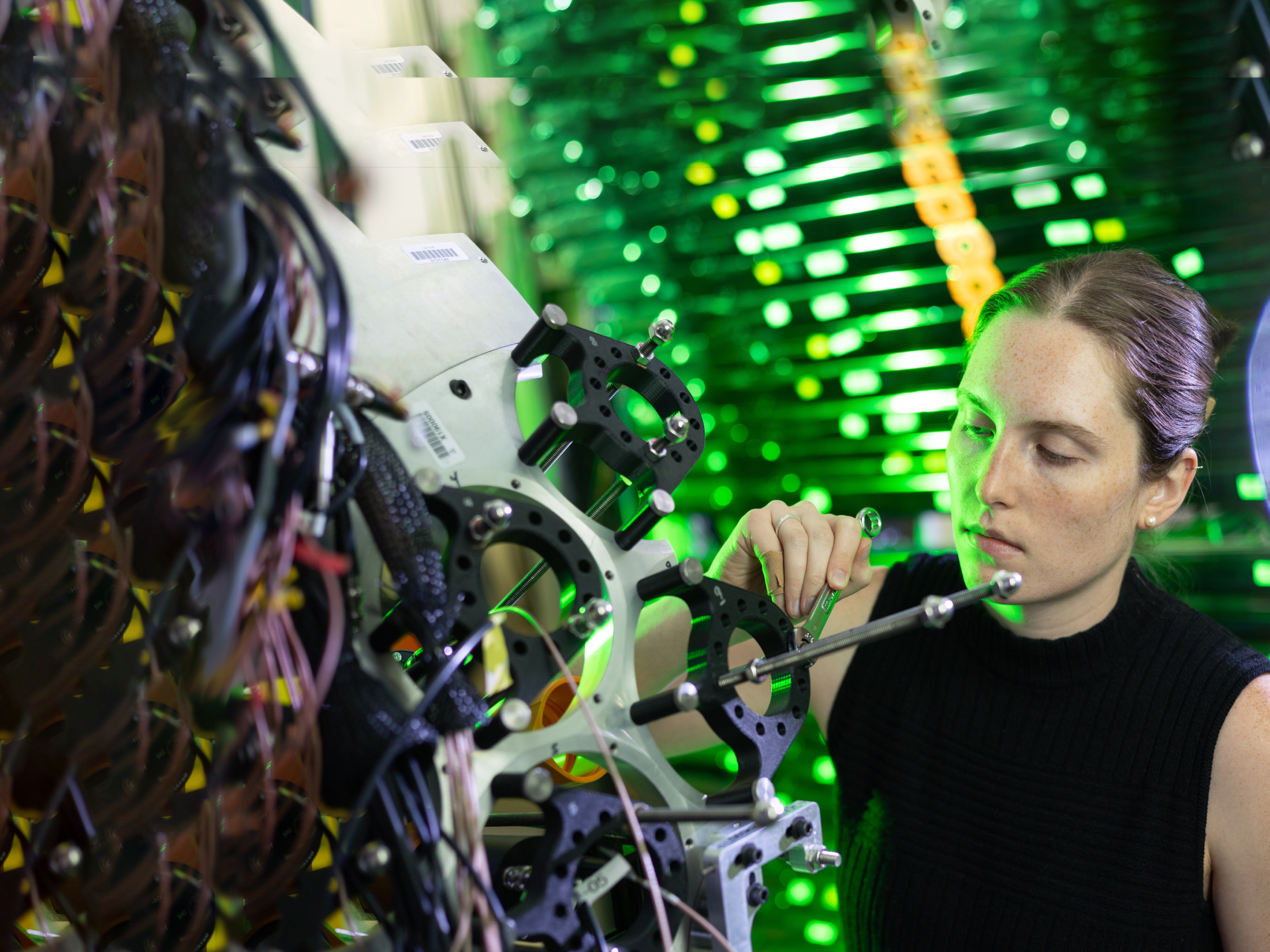
The Facility for Rare Isotope Beams (FRIB) at Michigan State University (MSU) is a world-class research and training center, hosting the most powerful rare isotope accelerator. MSU operates FRIB as a user facility for the U.S. Department of Energy Office of Science (DOE-SC), with financial support from and furthering the mission of the DOE-SC Office of Nuclear Physics. FRIB is where researchers come together to make discoveries that change the world. They study the properties and fundamental interactions of rare isotopes and nuclear astrophysics and their impact on medicine, homeland security, and industry.
FRIB advances nuclear science by improving our understanding of nuclei and their role in the universe, while also advancing accelerator systems.
In establishing and operating FRIB, capabilities were developed that transfer to other industries and applications.
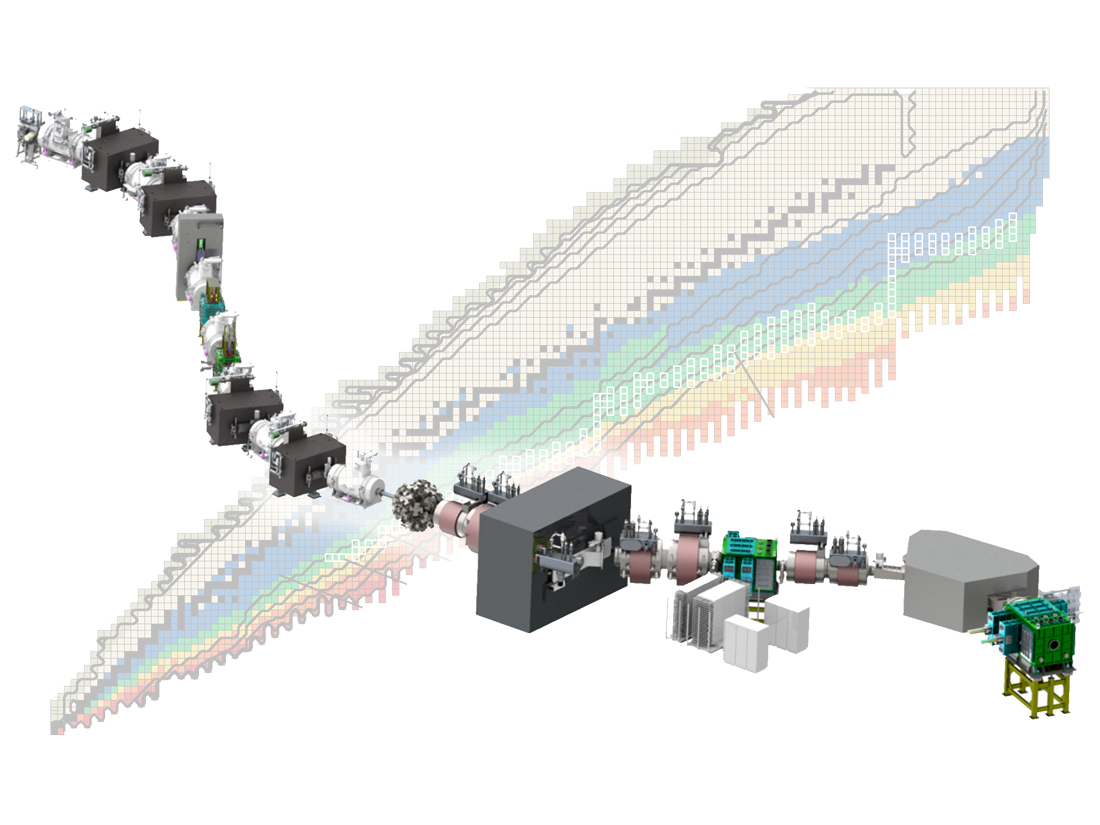
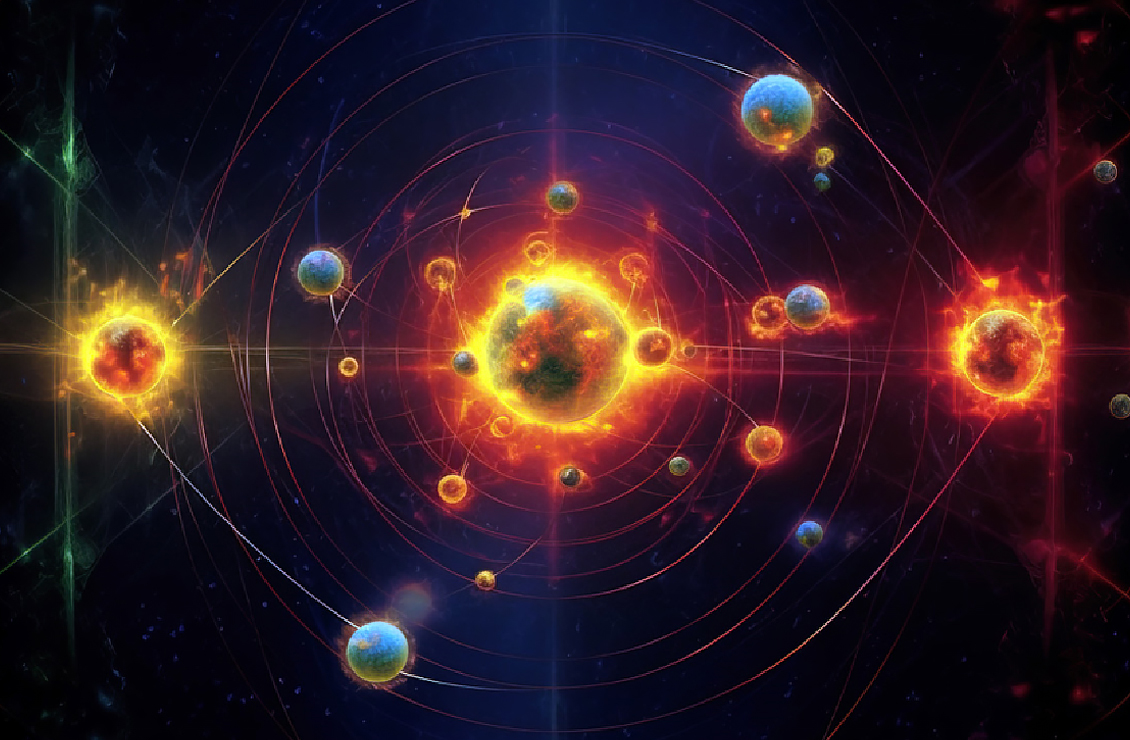
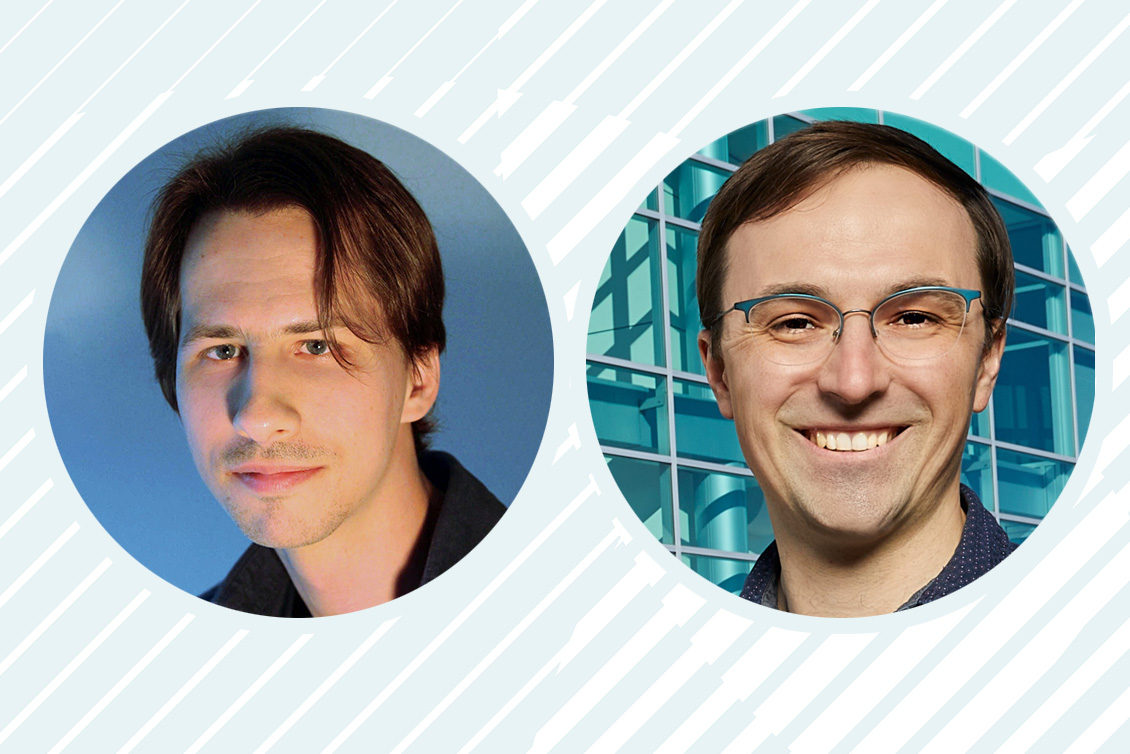
FRIB hosts the world’s most powerful heavy-ion accelerator and enables discoveries in rare isotopes, nuclear astrophysics, fundamental interactions, and societal applications like medicine, security, and industry.
Learn more about upcoming events taking place at FRIB.
Physics of Atomic Nuclei (PAN)
PAN introduces participants to the fundamentals of the extremely small domain of atomic nuclei and its connection to the extremely large domain of astrophysics and cosmology.
The PAN @ Michigan State Experience
- Learn about research in one of the top rare-isotope laboratories in the world.
- Get introduced to the fascinating fields of astrophysics, precision measurement, and nuclear science.
- Perform your own nuclear physics experiments.
- Meet researchers who are exploring a wide array of questions.
- Discover the surprising array of career opportunities in science.
- Experience the atmosphere of college life.
- Participants in the 2024 program get free room and board on campus (if required).
FRIB Theory Alliance Summer School - Emergence of Collective Motion in Atomic Nuclei
FRIB Theory Alliance Topical Program - Future directions in nuclear beta decay at FRIB
FRIB at MSU is a world-class research and training center where students and researchers from all career stages and backgrounds come together to make discoveries that change the world.
External news and journal publications discussing FRIB.
One of the nation's premier research facilities located at Michigan State University is getting a multi-million dollar upgrade. Late last month, the U.S. Department of Energy Office of Science approved $49.7 million for MSU's Facility for Rare Isotope Beams.
A team of scientists, including researchers from FRIB, published an article in Nature Physics on how research on neutron-rich nuclei shows that in the so-called islands of inversion, they are deformed rather than spherical in their ground states.
Scientists and engineers at the Facility for Rare Isotope Beams (FRIB) have reached a new milestone in isotope studies. They accelerated a high-power beam of uranium ions and delivered a record 10.4 kilowatts of continuous beam power to a target. The work is published in the journal Physical Review Accelerators and Beams.